Ever wanted to know a whole bunch of stuff about 3D bioprinting, its roots and limitations, but didn’t know where to turn? Professor Jordan Miller of the Department of Bioengineering at Rice University in Texas has published a pretty extensive (and open access) report on the role of 3D printing in organ transplantation and medicine at PLOS.
The report isn’t as long as Ulysses (though it does contain its fair share of footnotes), but it’s a bit too long to give adequate coverage here. Plus, it’s filled with all sorts of medical mumbo jumbo only doctors and scientists like to throw around, words like “subcutaneous”, “glycosaminoglycans”, and “blood”, so I’m not sure I would do it justice to clumsily break it all down here. There are some points that the report covers that would be pertinent to summarize however.
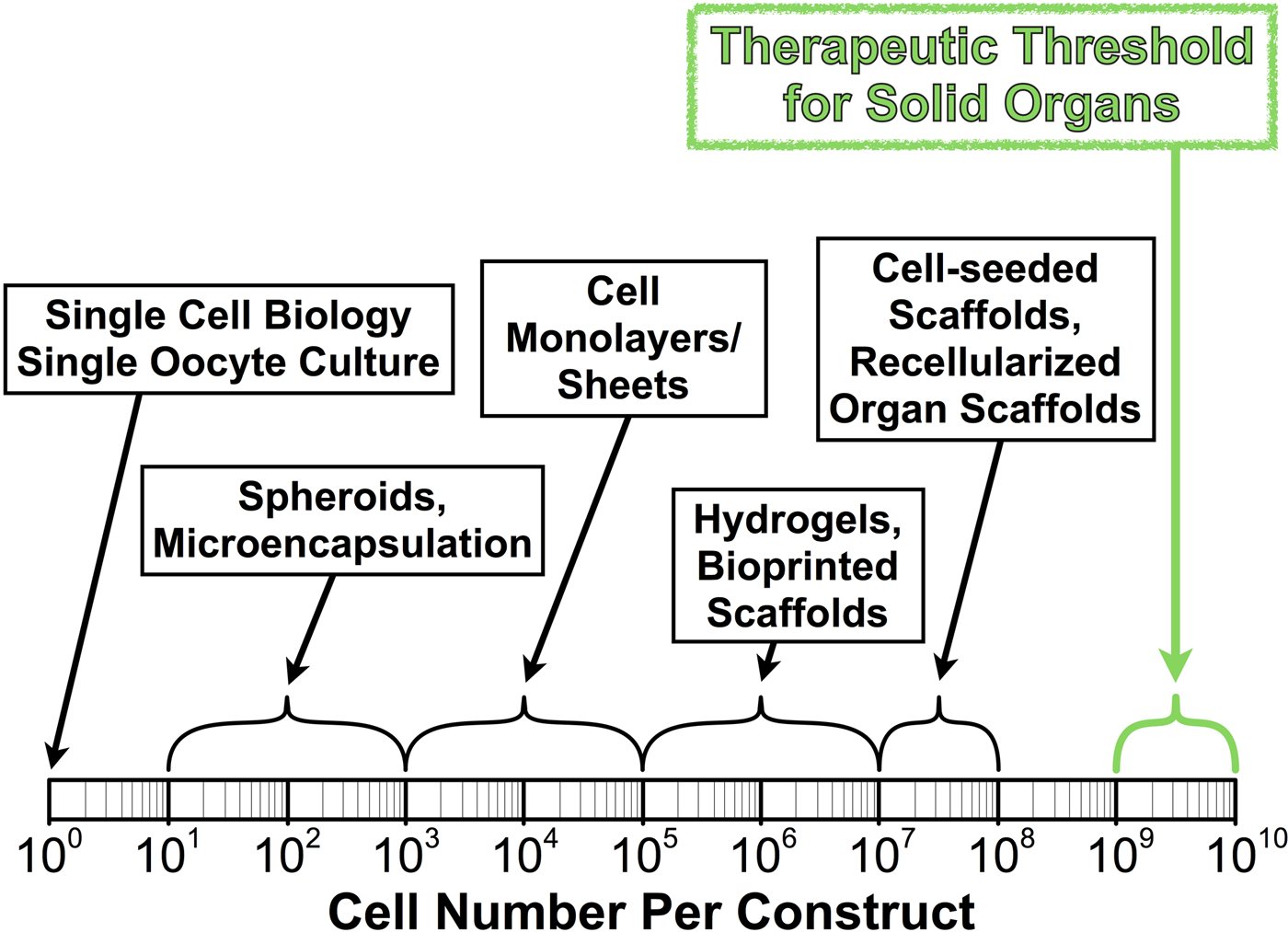
The report is titled “The Billion Cell Construct: Will Three-Dimensional Printing Get Us There?” because constructing a transplantable organ is a billion cell job. Miller describes the fact that, while we’ve been able to implant liver cells into rodents that spread and could perform the function of a whole liver, to do so in a human would mean drastically increasing the number of cells for a much larger organism. And, if we’re able to implant the 1-10 billion cells needed to perform an organ’s function, those cells will need to be structured with the appropriate architecture. You know what that means! 3D printing.
Right now, Miller points out that 3D printing biological matter has its problems. For instance, when using additive cellular inks, he writes, “some of the cell types most desirable for printing, such as hepatocytes [ed: liver cells], are actually quite fragile cells in culture; they may not survive the 3D printing process itself.” This issue might be resolved by some technical workarounds, such as “[j]ust-in-time cell harvesting, microfluidic culture devices, or automated cell sheet manipulation.” Miller hypothesizes that a combination of cellular lost wax casting, with “temporary inks can be printed, encased, and then selectively removed later”, and 3D printing could be the best approach to fabricating organs.
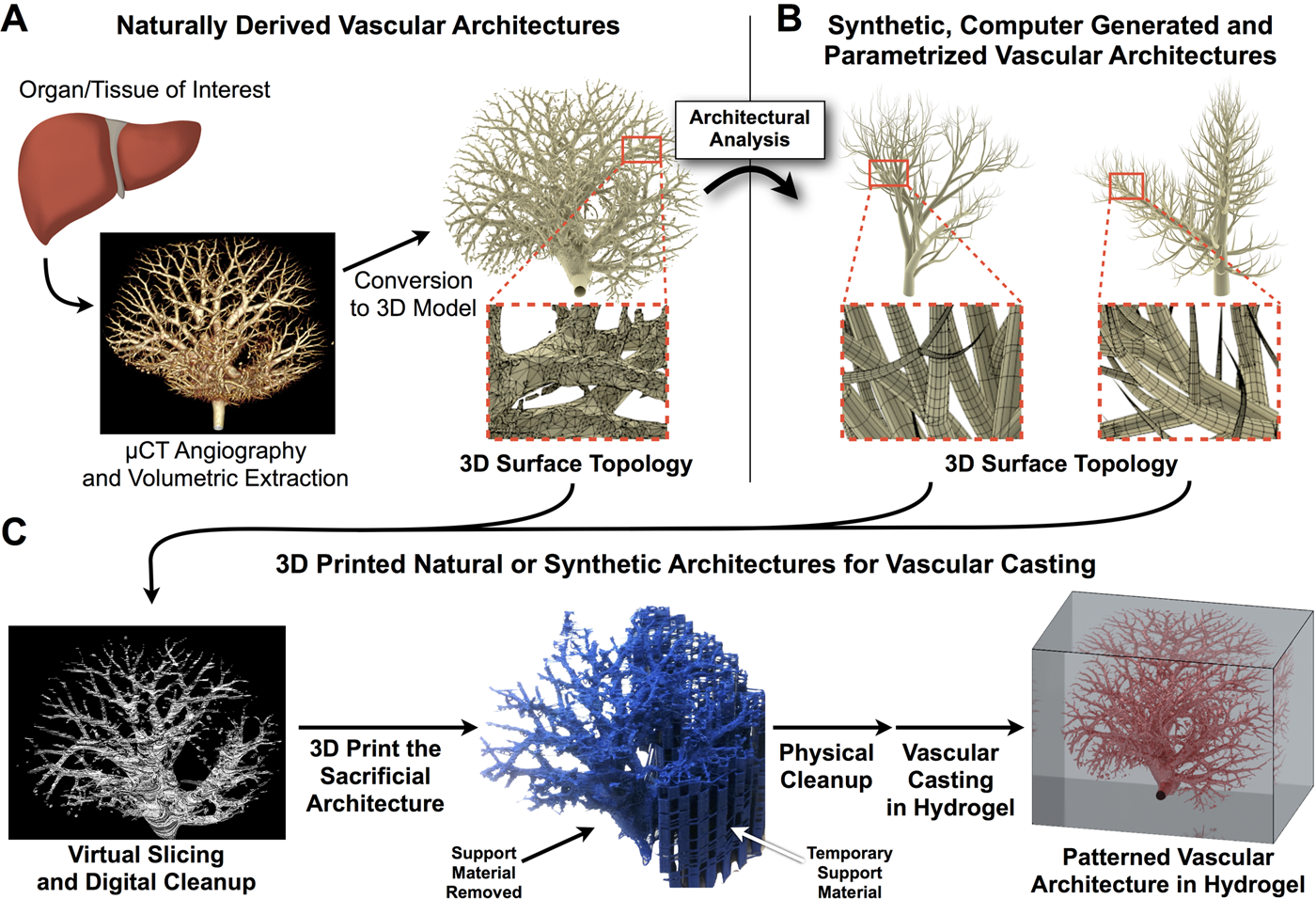
The author also gives an analysis of how, after we’re able to 3D print organs properly, to integrate them into the body of a patient. Hooking an organ transplant up to the circulatory system of a recipient would be difficult, but Miller believes that, by 3D printing organs made from “hydrogels containing living cells and perfusable vasculature” it might help bypass some of the problems associated with transplanting an organ into a host’s vascular system.
As far as attaching 3D printed organs into a patient’s nervous system, Miller doesn’t see that as much of a problem, saying, “[The] neurovascular junction probably isn’t critical for initial attempts to synthesize living tissue. Transplanted human hearts, for example, are not surgically tied to the nerves of the recipient; they beat at their own pace. And proximal sensory nerves can innervate and restore feeling in regenerated skin substitutes. So it may be that such re-innervation will work in other engineered organ systems.”
Once we get the hang of it, Miller lists a number of uses for 3D bioprinting, ranging from pharmaceutical testing, such as the work that Organovo is pursuing with their 3D printed arrays of liver cells, to understanding the progression of disease. Researchers could potentially 3D print cellular arrays, mimicking the natural environment of the human body, with which to predict the effects of chemotherapy.
What’s best about this report, aside from all of the fun bioprinting stuff, is that Miller is a big advocate of the open source movement. By making this report open access, he is inviting readers outside of the medical and scientific community to join in the dialogue about 3D printing and organ transplantation. It’s no surprise that he’s an advocate of open source, either, because Prof. Miller is also one of the faculty members behind the Advanced Manufacturing Research Institute, also based out of Rice, or RepRap U, as I like to call it. RepRap U is responsible for such projects as this open source laser sintering printer. You may be more familiar with his work with Penn, where he and his team were responsible for 3D printing the vascular structures of organs with sugar.
If there is a god, then the next big open source movement to take place will be 3D bioprinting.